Reading the Layers of Time
Ice cores, drilled from glaciers and ice sheets in places like Antarctica and Greenland, are invaluable archives of Earth's past climate and atmosphere. They form as layers of snow accumulate year after year, compressing under their own weight into solid ice. Each layer traps information about the environment at the time it formed.
Annual Layers
Similar to tree rings, distinct annual layers can often be identified in the upper sections of ice cores. These layers arise from seasonal variations in snowfall characteristics:
- Summer snow: Often lighter, less dense, potentially containing melt layers or different crystal structures.
- Winter snow: Denser, sometimes containing more wind-blown dust or different chemical impurities.
- Isotopic Composition: The ratio of heavy to light isotopes of oxygen (¹⁸O/¹⁶O) and hydrogen (²H/¹H) in the ice varies with temperature, showing seasonal cycles.
These variations allow scientists to count back years, layer by layer.
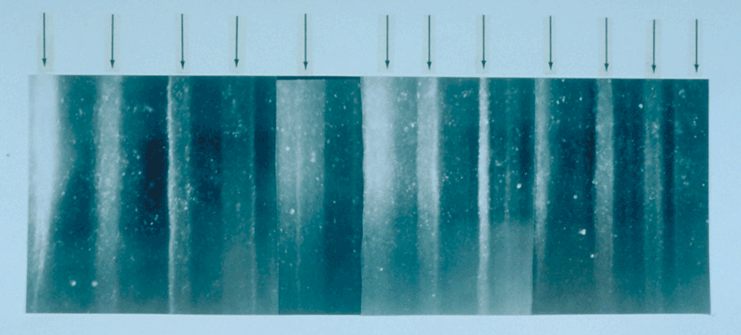
Methodology: Drilling and Analysis
Obtaining and dating ice cores is a complex process involving specialized techniques:
Sophisticated drills are used to extract cores, often several kilometers long, from the ice sheet without fracturing or contaminating them. Drilling fluid is sometimes used to keep the borehole open at great depths.
Cores are meticulously logged, cut, and analyzed in cold laboratories. Techniques include visual stratigraphy, electrical conductivity measurements (ECM), chemical analysis (ions, isotopes), dust particle counting, and gas analysis from trapped air bubbles.
Continuous Melter Systems
Modern techniques often involve continuously melting the core and feeding the meltwater into various instruments for high-resolution analysis of its chemical and physical properties along its length.
Counting the Years: Annual Layer Identification
Directly counting annual layers is the most accurate dating method for the upper, less compacted parts of an ice core. Several indicators are used, often in combination:
- Visual Stratigraphy: Observing changes in ice texture, bubble density, and visible dust layers corresponding to seasonal changes.
- Electrical Conductivity/Dielectric Profiling (ECM/DEP): These methods detect changes in ice chemistry, particularly acidity spikes from volcanic eruptions or seasonal ion variations, which help define layer boundaries.
- Chemical Signatures: Seasonal cycles in the concentration of chemical species like sea salts (sodium, chloride), dust (calcium, potassium), ammonium, nitrate, and stable water isotopes (δ¹⁸O, δD) provide annual markers.
Decreasing Resolution with Depth
As depth increases, the ice layers become compressed and thinned due to the immense pressure and the slow flow of the ice sheet. Eventually, annual layers become too thin to distinguish reliably, typically beyond tens of thousands of years, requiring other dating methods.
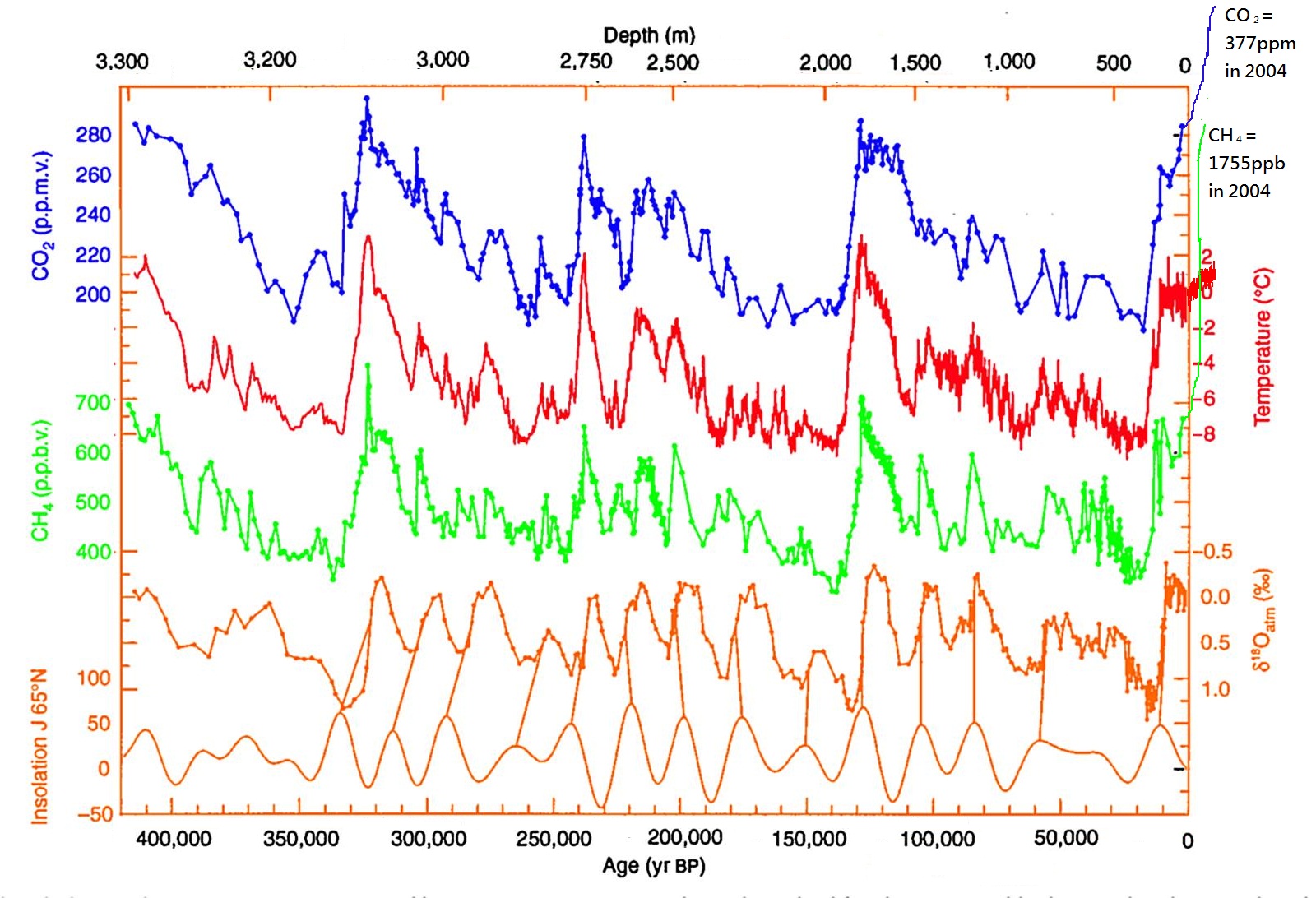
Anchoring the Timescale: Reference Horizons
Where annual layers become indistinct, scientists rely on identifying globally or regionally synchronous "marker" layers within the ice whose age is known from other sources.
1. Volcanic Eruptions (Tephrochronology)
Major volcanic eruptions eject ash (tephra) and sulfur dioxide into the stratosphere, leading to widespread deposition. These result in visible ash layers and significant sulfate spikes in ice cores globally. If the eruption date is known historically or from other geological records, these layers provide precise time markers.
2. Cosmogenic Isotopes
Cosmogenic Isotopes like Beryllium-10 (¹⁰Be) and Carbon-14 (¹⁴C, measured in trapped CO₂) are produced in the atmosphere by cosmic rays. Their production rate varies with solar activity and Earth's magnetic field. Known peaks or events (e.g., the Laschamp geomagnetic excursion ~41,000 years ago, major solar proton events) can be identified in the ice core and used as time markers.
3. Abrupt Climate Events
Rapid climate shifts recorded in multiple paleoclimate archives (e.g., Dansgaard-Oeschger events, Heinrich events) can sometimes be identified in the ice core chemistry or dust content and used for correlation and dating, although their synchronicity needs careful evaluation.
Modeling Ice Flow
Beneath the zone where annual layers can be counted, the age-depth relationship is primarily determined using ice flow models. These mathematical models simulate how ice accumulates, compacts, and flows under pressure from the surface towards the margins or base of the ice sheet.
How Models Work
Models incorporate factors like:
- Surface accumulation rate (which may have varied in the past).
- Ice thickness.
- Basal conditions (temperature, meltwater).
- Ice rheology (how ice deforms under stress).
- Geometry of the bedrock beneath the ice.
By calculating how much layers thin as they are buried and flow outwards, models estimate the age at any given depth. They are constrained and validated using the known ages and depths of reference horizons (like volcanic layers) and the total depth/age at the bottom if known.
Combining Methods
Ice core chronologies are typically constructed using a combination of annual layer counting in the upper sections, identification of reference horizons, and ice flow modeling for the deeper, older ice. The consistency between these independent approaches provides confidence in the resulting timescale.
Other Dating Techniques
Several other methods complement layer counting and flow modeling:
Radiometric Dating
- Carbon-14 (¹⁴C) Dating: The ¹⁴C content of CO₂ trapped in air bubbles can be measured. However, this is challenging due to the small sample size and potential contamination. It's primarily used as a consistency check or for specific intervals rather than the primary dating method.
- Argon Dating (⁴⁰Ar/³⁹Ar): Volcanic ash layers trapped within the ice can sometimes be dated using the Argon-Argon method, providing absolute age points for the chronology.
- Other Isotopes: Methods involving Krypton-81 (⁸¹Kr) or Chlorine-36 (³⁶Cl) in trapped air or ice are being developed for dating very old ice.
Orbital Tuning
For very long cores extending back hundreds of thousands of years, chronologies can be refined by correlating climate cycles observed in the ice core (e.g., derived from isotopic temperature proxies or greenhouse gas concentrations) with calculated variations in Earth's orbit (Milankovitch cycles), which are known to pace ice ages. This method, called orbital tuning, helps date the oldest parts of the record where other methods have large uncertainties.
Applications: Windows into the Past
Dated ice cores provide unparalleled insights into past environmental conditions:
Paleoclimate Reconstruction
Stable isotopes (δ¹⁸O, δD) act as proxies for past temperature. Dust concentration reflects atmospheric circulation and aridity. Chemical composition reveals information about sea ice extent, biological activity, and atmospheric transport.
Past Atmospheric Composition
Trapped air bubbles provide direct samples of past air, allowing measurement of greenhouse gases (CO₂, CH₄, N₂O), revealing natural climate forcings and the context for current anthropogenic changes.
Volcanic History & Environmental Events
Records of past volcanic eruptions, large wildfires (from soot), biomass burning events, and atmospheric pollution.
Long-Term Records
Cores like EPICA Dome C in Antarctica extend back over 800,000 years, covering multiple glacial-interglacial cycles and providing crucial data for understanding long-term climate dynamics and the Earth system.
Limitations and Uncertainties
Ice core dating, while powerful, has inherent limitations and uncertainties:
Layer Thinning & Diffusion
Annual layers become extremely thin at depth, making them impossible to resolve. Diffusion processes can smooth out sharp chemical or isotopic signals over long timescales, especially in warmer ice near the bedrock.
Ice Flow Complexity
Accurate ice flow modeling can be challenging, especially near the bed where flow can be complex or where basal melting occurs. Uncertainties in past accumulation rates also affect model accuracy.
Gas Age vs. Ice Age
Air bubbles only become sealed off from the atmosphere deep in the firn (unconsolidated snow) layer, typically tens to hundreds of meters below the surface. Therefore, the air trapped in bubbles is younger than the surrounding ice (the "delta age"). This difference must be carefully estimated, adding uncertainty.
Potential Discontinuities
Ice flow abnormalities, basal melting, or past surface conditions could potentially lead to disturbed or missing sections (hiatuses) in the record, especially near the bottom of the ice sheet.
Geographic Limitation
High-resolution, long ice cores can only be obtained from thick, cold, stable ice sheets or glaciers, primarily in polar regions (Antarctica, Greenland) and some high-mountain areas.
Conclusion: A Robust Multiproxy Chronometer
Ice core dating relies on a combination of methods, each with its strengths and weaknesses, providing robust chronologies extending back hundreds of thousands of years. Its reliability stems from:
- Direct Layer Counting: Providing annual resolution where possible.
- Cross-Validation: Using multiple indicators (visual, chemical, electrical) to identify annual layers.
- Independent Time Markers: Anchoring the timescale with datable reference horizons like volcanic ash.
- Physics-Based Modeling: Using well-understood principles of ice flow, constrained by data.
- Consistency Checks: Comparing results with other dating methods (radiometric, orbital tuning) and independent paleoclimate records.
Ice cores offer an exceptionally detailed glimpse into past climate and atmospheric changes, providing critical context for understanding Earth's climate system and the impact of human activities.